Polo-like kinase 1 inhibitors in refractory colorectal cancer: deciphering the myth of synthetic lethality
Metastatic colorectal cancer is a dismal disease because, despite initial sensitivity to chemotherapy and targeted therapies [anti-vascular endothelial growth factor (anti-VEGF) and anti-epidermal growth factor receptor (anti-EGFR)], treatment resistance rapidly arises and becomes universal. Consequently, second-line therapy with chemotherapy and bevacizumab exhibits poor efficacy in RAS mutant metastatic colorectal cancer patients who progress on first-line therapy, with response rates ranging between 10% and 15% and progression-free survivals (PFSs) of 6 to 7 months, depending on previous bevacizumab exposure (1,2). The mechanisms underlying chemotherapy and bevacizumab resistance remain unclear, and there are accordingly no customized second-line therapeutic strategies for advanced colorectal cancer. The key players linked to chemotherapy resistance in advanced colorectal cancer include oxidative phosphorylation (OXPHOS), a main metabolic program in cancer (3), glucose-6-phosphate dehydrogenase, a critical enzyme in the antioxidant-generating pentose phosphate pathway (PPP) (4), and polo-like kinase-1 (PLK1) (5), an enzyme mainly implicated in cell cycle regulation. How these critical oncological pathways participate in acquired resistance after initial therapy and how they cooperate to sustain this resistance are currently unknown.
In a recent issue of Clinical Cancer Research, Ahn and collaborators present the results of a phase Ib clinical trial in 18 RAS mutant patients who received second-line therapy with FOLFIRI + bevacizumab + onvansertib (a PLK1 inhibitor) with an impressive response rate (44%) and a promising median PFS of 12.6 months [95% confidence interval (CI), 9.34–not reached] and median duration of response of 9.5 months (95% CI, 8.9–not reached) (6). In addition, two patients achieved durable stable disease (progression at 21 and 25 months from study entry). The authors selected KRAS mutant tumors based on very limited pre-clinical studies that showed better efficacy in DLD1 isogenic KRAS mutant compared with KRAS wild-type and in a unique HCT116 KRAS mutant xenograft model. Notably, an ongoing prospective phase II randomized trial is comparing FOLFIRI + bevacizumab versus FOLFIRI + bevacizumab + onvansertib in pretreated RAS mutant colorectal cancer patients (NCT05593328).
Our previous work showed that tumors display two major metabolic pathways, glycolytic and OXPHOS (7). Patients with the OXPHOS phenotype (roughly 60–70% of tumors) are more dependent on glutamine and fatty acids than glycolytic tumors and exhibit the concomitant activation of multiple antioxidant pathways [e.g., PPP, isocitrate dehydrogenase (IDH), folate, and glutamine/cysteine exchange (xCT)]. In addition, OXPHOS tumors have increased chromosome instability (CIN) and mitotic cell cycle markers (E2F and G2M) and decreased immune and fibroblast infiltration compared with glycolytic tumors.
BRCAness tumors (BRCA1/2 and PALB2 mutations and/or RAD51 deficiency) with loss of homologous recombination (HR) function are more PARP dependent and, interestingly, rely on OXPHOS instead of glycolysis (8). These tumors are more sensitive to PARP inhibition with olaparib than glycolytic tumors, although the combination of OXPHOS inhibitors and PARP inhibitors did not show synergy. The authors attributed the absence of synergy between OXPHOS and PARP inhibitors to the shift from OXPHOS to glycolysis after OXPHOS inhibition. Importantly, BRCAness-independent tumors with abundancy of specific metabolites such as 2-hydroxiglutarate, fumarate, and succinate due to mutations in IDH, fumarate dehydrogenase (FH), and succinate dehydrogenase (SDH) can also possess impaired HR functionality and therefore be potentially more sensitive to PARP inhibitors (9). Although mutations in IDH, SDH, and FH are extremely rare in colorectal cancer, altered SDH levels have been reported (10). In acute myeloid leukemia with FLT3 internal tandem duplications, which are tumors that basally rely on OXPHOS, we previously determined that SDH inhibition results in the use of lactate as fuel for mitochondria respiration; this can be exploited therapeutically by blocking monocarboxylic acid transporter (MCT1) (11). We must note that these tumors still rely on OXPHOS (i.e., are not glycolytic) because the lactate is used to fuel the electron transport chain in the mitochondria. Moreover, MCT1 has also been shown to increase the PPP, a process that is required to induce bulky metastatic tumors (12). In addition, because PLK1 also activates glucose-6-phosphate dehydrogenase, a key enzyme in the PPP (13), we hypothesize that these tumors, after OXPHOS inhibition, retain the OXPHOS phenotype but acquire the ability to use atypical nutrients (lactate probably) to fulfill both bulky requirements (and become more aggressive) and antioxidant mechanisms of defense (and become more resistant). Despite a rational for the use of PARP inhibitors alone or in combination with oxaliplatin in BRCAness colorectal pre-clinical models (14) no significant benefit of olaparib was found in unselected oxaliplatin-refractory BRCAness patients (15) and as a maintenance strategy in oxaliplatin-sensitive patients (16).
The lack of clinical olaparib efficacy in colorectal cancer could reflect the loss of a major role for HR and non-homologous end-joining (NHEJ) in BRCAness and non-BRCAness high-CIN tumors early after FOLFOX therapy. A prominent function for PLK1 seems to be in controlling another cell cycle checkpoint, microhomology-mediated end-joining (MMEJ), which adequately repairs DNA damage in mitosis in tumors with persistent double-strand breaks that exhibited previous HR-mediated DNA repair failure (in S and G2 phases) or that of NHEJ (in G1 phase) (17). PLK1 is a serine-threonine kinase that is overexpressed in colorectal cancer. Nonetheless, its function can also be impaired in heavily pretreated colorectal cancer patients, facilitating CIN instead of adequate DNA repair and consequently allowing double-strand breaks to persist throughout duplication and mitosis, increasing CIN. Indeed, it has recently been shown that loss of ubiquitin-binding protein-2-like (UBAP2L) can increase PLK1 expression and lead to the accumulation of genome errors and CIN (18).
Returning to the study by Ahn et al., we must point out some limitations. First, just 44% of their patients responded to the therapy and only two patients seemed to obtain a long-term benefit. Therefore, more than 50% of patients had intrinsic resistance and most of the initially sensitive patients acquired resistance over time. In patients with the OXPHOS phenotype, the concomitant presence of an antioxidant pathway can restore cancer cell resistance to synthetic lethality by reducing the antitumor efficacy of reactive oxygen species, thereby linking OXPHOS, PPP, and PLK1 to acquired chemoresistance in colorectal cancer. Second, the authors selected patients with RAS mutant tumors, even though colorectal cancer patients with BRAF mutation and double wild-type genotype can also exploit OXPHOS metabolism. Third, glycolytic tumors or tumors with OXPHOS that do not use PPP as a main antioxidant buffer pathway would be intrinsically resistant to onvansertib.
Therefore, expanding the patient selection (patients with OXPHOS and PPP as the main antioxidant pathway) could be a strategy to improve onvansertib activity. Second, the design of new optimized combinations may also increase the clinical efficacy of onvansertib therapy. Because both DNA repair mechanisms (MMEJ in mitosis and HR/NHEJ in interphase) seem important in metastatic colorectal cancer patients (either in BRCAness and non-BRCAness patients and high-CIN patients), there is a rational for combining PLK1 (or polymerase theta or TOPB1) inhibitors with PARP inhibitors, which have shown good efficacy in pre-clinical models (19). Several recent pre-clinical studies have demonstrated that double-strand breaks increase extracellular cGAMP levels but induce heavy immunosuppression in tumors with high ENPP1 expression due to decreased STING activation in immune cells such as macrophages, myeloid-derived suppressor cells, and dendritic cells and an increased adenosine presence (20,21). Therefore, a combination of ENPP1 inhibitors with programmed cell death protein 1 (PD-1) inhibitors has been proposed to increase anti-PD-1 efficacy based on pre-clinical models. However, we have to note that none of these pre-clinical studies used models of pretreated microsatellite stable colorectal cancer. PLK1 is also implicated in the direct activation of MYC (22). Because MYC is a well-known immunosuppressive inducer (23), the combination of PLK1 and ENPP1 inhibitors with immune checkpoint blockade therapy seems to be a promising clinical approach in tumors that rely on high CIN and high immunosuppression, such as heavily pretreated microsatellite stable colorectal cancer. In Figure 1, we stated multiple mechanisms associated to chemotherapy and immune-resistance in OXPHOS tumors.
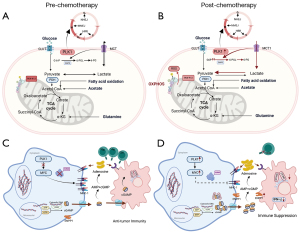
Ongoing clinical trials across various tumor types (KEYLYNK-007-NCT04123366) are currently evaluating the efficacy of olaparib + pembrolizumab in initially oxaliplatin-sensitive BRCAness patients and onvansertib in second-line therapy in RAS mutant patients after oxaliplatin progression (NCT05593328). We should emphasize that, if these studies do not achieve significantly positive outcomes, there is still room for improvement by pre-selecting patients with enhanced OXPHOS and PPP and optimizing drug combinations.
Acknowledgments
Funding: None.
Footnote
Provenance and Peer Review: This article was commissioned by the editorial office, AME Clinical Trials Review. The article has undergone external peer review.
Peer Review File: Available at https://actr.amegroups.com/article/view/10.21037/actr-24-68/prf
Conflicts of Interest: All authors have completed the ICMJE uniform disclosure form (available at https://actr.amegroups.com/article/view/10.21037/actr-24-68/coif). J.M. has received grants to institution from Instituto de Salud Carlos III (IP FIS19-PI19/00740), and support from AMGEM for financial travel and congress; has served on the Advisory Boards of Advance Medical, Cancer Expert Now, MSD, Delcath Systems, Inc.; has a leadership role in GEMCAD, and also has non-financial interests with Amgen, Guardant, Incyte, Merck, Terumo, CIBEREHD. The other authors have no conflicts of interest to declare.
Ethical Statement: The authors are accountable for all aspects of the work in ensuring that questions related to the accuracy or integrity of any part of the work are appropriately investigated and resolved.
Open Access Statement: This is an Open Access article distributed in accordance with the Creative Commons Attribution-NonCommercial-NoDerivs 4.0 International License (CC BY-NC-ND 4.0), which permits the non-commercial replication and distribution of the article with the strict proviso that no changes or edits are made and the original work is properly cited (including links to both the formal publication through the relevant DOI and the license). See: https://creativecommons.org/licenses/by-nc-nd/4.0/.
References
- Giantonio BJ, Catalano PJ, Meropol NJ, et al. Bevacizumab in combination with oxaliplatin, fluorouracil, and leucovorin (FOLFOX4) for previously treated metastatic colorectal cancer: results from the Eastern Cooperative Oncology Group Study E3200. J Clin Oncol 2007;25:1539-44. [Crossref] [PubMed]
- Bennouna J, Sastre J, Arnold D, et al. Continuation of bevacizumab after first progression in metastatic colorectal cancer (ML18147): a randomised phase 3 trial. Lancet Oncol 2013;14:29-37. [Crossref] [PubMed]
- Vellinga TT, Borovski T, de Boer VC, et al. SIRT1/PGC1α-Dependent Increase in Oxidative Phosphorylation Supports Chemotherapy Resistance of Colon Cancer. Clin Cancer Res 2015;21:2870-9. [Crossref] [PubMed]
- Ju HQ, Lu YX, Wu QN, et al. Disrupting G6PD-mediated Redox homeostasis enhances chemosensitivity in colorectal cancer. Oncogene 2017;36:6282-92. [Crossref] [PubMed]
- Yu Z, Deng P, Chen Y, et al. Inhibition of the PLK1-Coupled Cell Cycle Machinery Overcomes Resistance to Oxaliplatin in Colorectal Cancer. Adv Sci (Weinh) 2021;8:e2100759. [Crossref] [PubMed]
- Ahn DH, Barzi A, Ridinger M, et al. Onvansertib in Combination with FOLFIRI and Bevacizumab in Second-Line Treatment of KRAS-Mutant Metastatic Colorectal Cancer: A Phase Ib Clinical Study. Clin Cancer Res 2024;30:2039-47. [Crossref] [PubMed]
- Pedrosa L, Foguet C, Oliveres H, et al. A novel gene signature unveils three distinct immune-metabolic rewiring patterns conserved across diverse tumor types and associated with outcomes. Front Immunol 2022;13:926304. [Crossref] [PubMed]
- Lahiguera Á, Hyroššová P, Figueras A, et al. Tumors defective in homologous recombination rely on oxidative metabolism: relevance to treatments with PARP inhibitors. EMBO Mol Med 2020;12:e11217. [Crossref] [PubMed]
- Sulkowski PL, Oeck S, Dow J, et al. Oncometabolites suppress DNA repair by disrupting local chromatin signalling. Nature 2020;582:586-91. [Crossref] [PubMed]
- Nan H, Guo P, Fan J, et al. Comprehensive analysis of the prognosis, tumor microenvironment, and immunotherapy response of SDHs in colon adenocarcinoma. Front Immunol 2023;14:1093974. [Crossref] [PubMed]
- Erdem A, Marin S, Pereira-Martins DA, et al. Inhibition of the succinyl dehydrogenase complex in acute myeloid leukemia leads to a lactate-fuelled respiratory metabolic vulnerability. Nat Commun 2022;13:2013. [Crossref] [PubMed]
- Tasdogan A, Faubert B, Ramesh V, et al. Metabolic heterogeneity confers differences in melanoma metastatic potential. Nature 2020;577:115-20. [Crossref] [PubMed]
- Ma X, Wang L, Huang D, et al. Polo-like kinase 1 coordinates biosynthesis during cell cycle progression by directly activating pentose phosphate pathway. Nat Commun 2017;8:1506. [Crossref] [PubMed]
- Arena S, Corti G, Durinikova E, et al. A Subset of Colorectal Cancers with Cross-Sensitivity to Olaparib and Oxaliplatin. Clin Cancer Res 2020;26:1372-84. [Crossref] [PubMed]
- Leichman L. Going Back. Oncologist 2021;26:86-7. [Crossref] [PubMed]
- Takashima A, García-Alfonso P, Manneh R, et al. Olaparib with or without bevacizumab versus bevacizumab plus a fluoropyrimidine as maintenance therapy in advanced colorectal cancer: The randomized phase 3 LYNK-003 study. Eur J Cancer 2024;205:114036. [Crossref] [PubMed]
- Brambati A, Sacco O, Porcella S, et al. RHINO directs MMEJ to repair DNA breaks in mitosis. Science 2023;381:653-60. [Crossref] [PubMed]
- Guerber L, Vuidel A, Liao Y, et al. UBAP2L-dependent coupling of PLK1 localization and stability during mitosis. EMBO Rep 2023;24:e56241. [Crossref] [PubMed]
- Zhou J, Gelot C, Pantelidou C, et al. A first-in-class Polymerase Theta Inhibitor selectively targets Homologous-Recombination-Deficient Tumors. Nat Cancer 2021;2:598-610. [Crossref] [PubMed]
- Li J, Duran MA, Dhanota N, et al. Metastasis and Immune Evasion from Extracellular cGAMP Hydrolysis. Cancer Discov 2021;11:1212-27. [Crossref] [PubMed]
- Wang S, Böhnert V, Joseph AJ, et al. ENPP1 is an innate immune checkpoint of the anticancer cGAMP-STING pathway in breast cancer. Proc Natl Acad Sci U S A 2023;120:e2313693120. [Crossref] [PubMed]
- Tan J, Li Z, Lee PL, et al. PDK1 signaling toward PLK1-MYC activation confers oncogenic transformation, tumor-initiating cell activation, and resistance to mTOR-targeted therapy. Cancer Discov 2013;3:1156-71. [Crossref] [PubMed]
- Demel UM, Böger M, Yousefian S, et al. Activated SUMOylation restricts MHC class I antigen presentation to confer immune evasion in cancer. J Clin Invest 2022;132:e152383. [Crossref] [PubMed]
Cite this article as: Rojas M, Gonzalez L, Cascante M, Maurel J. Polo-like kinase 1 inhibitors in refractory colorectal cancer: deciphering the myth of synthetic lethality. AME Clin Trials Rev 2024;2:48.